Graphene nanoribbons with zigzag edges (Z-GNRs) have attracted intense attention owing to their unique properties arising from the edges. Many theoretical and experimental investigations have shown that they are metallic, with peculiar edge states on both edges of the ribbon, regardless of their width. Such Z-GNRs are predicted to be able to carry a spin current around the edge with dangling bonds, which makes them potentially applicable for nanoscale spintronic devices. Since the ribbon’s edge plays an important role in determination of Z-GNRs’ properties, an insightful understanding of the phonon dispersion at the edges is essential.
Previously, the research groups led by Prof. ZHANG guangyu in the Institute of Physics, Chinese Academy of Sciences (IOP) and Beijing National Laboratory for Condensed Matter Physics reported a series of advances in graphene nanostructure fabrication techniques. They reported a highly anisotropic dry-etching technique in graphene basal plane for the first time [Advanced Materials 22, 4014, (2010)]. They also creatively combine this technique with artificial-defect engineering developed a well-controlled top-down approach to fabricate zigzag-edged graphene nanostructures with well-controlled widths [Advanced Materials 23, 3061, (2011)].
Recently, they have systematically studied the Raman scattering for Z-GNRs. They observed the G-peak split that is due to a TO phonon of the G-line (G
--1583) in Z-GNRs softened by localized electron screening for the first time. The relative intensity ratio of G
-/G
+ is highly dependent on the nanoribbon width, with a critical ribbon width of ~5(?3) nm, under which only the G- peak is present and the G
+ totally disappears. They attribute the G-splitting to zigzag edges, so the appearance of the characteristic G
- peak can be used as a fingerprint peak for Z-GNRs. Moreover, the four-fold symmetry of the G-peak (degenerated TO mode, for both G
- and G
+) for Z-GNRs were also confirmed experimentally by the polarized Raman spectra for the first time. Their results here provide a first and direct experimental study on the vibrational properties of Z-GNRs. This research result has been published in Nano Letters 11, 4083 (2011).
This work was supported by NSFC and National 973 projects.
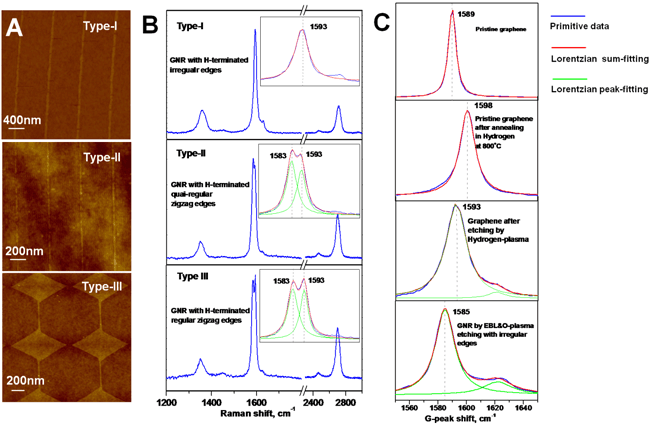 |
Figure 1Three types of GNRs with different edges. (A) AFM images of three types of graphene nanoribbons with different edges: totally disordered edge (type I), zigzag-enriched edge (type II), and nearly pure zigzag edge (type III). (B) Typical Raman spectra for these GNRs with the insets of zoom-in spectra for G-peaks in the upper right. (C) G-peaks comparison for pristine graphene, graphene annealed in hydrogen at 800oC, graphene etched in hydrogen-plasma and oxygen-terminated GNRs. (Image by ZHANG guangyu et al.) |
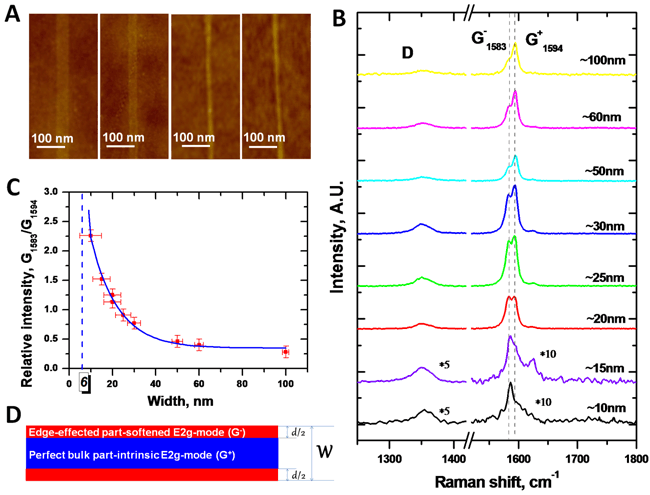 |
Figure 2Width dependence of the relative intensity G-/G+. (A) AFM images of Type-III GNRs at different widths after nanopads removing. (B) Raman spectra for these Z-GNRs at different widths. (C) Fitted width dependence of the relative intensity G-/G+. (D) Schematic of two-component Z-GNR: edge affected part (softened E2g-mode G-as shown in red) and perfect bulk part (intrinsic E2g-mode G+as shown in blue). (Image by ZHANG guangyu et al.) |
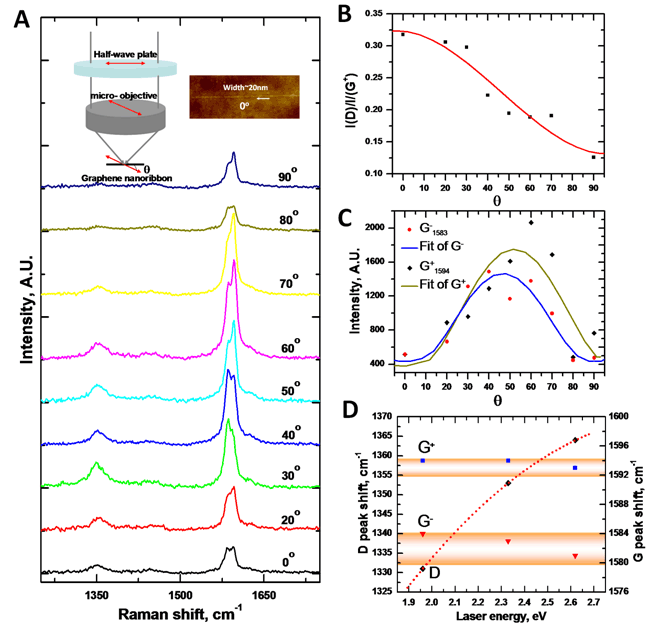 |
Figure 3Polarized Raman scattering of Type-II GNRs. (A) Raman spectra of a Z-GNR (width ~ 20nm, as shown in the inset AFM image) in the VV configuration for various angles between the Z-GNR axis and the polarization of the incident laser beam, as depicted in the inset. (B) The relative intensity I(D)/I(G+) as a function of ?. (C) Intensity of G-and G+peaks versus ?. The lines are fitted curves. (D) Raman frequencies of the D, G-and G+peaks as a function of the laser energy. (Image by ZHANG guangyu et al.) |