It is known to all that the DNA molecule is the carrier of genetic code for all living organisms. Because of its unique self-recognition and self-assembling properties, the DNA molecule is regarded as a promising candidate for molecular electronics, and its charge transport property has attracted considerable attention among physics, chemistry, and biology communities during the last two decades. Recently, the spin selectivity of photoelectron transmission through self-assembled monolayers of double-stranded DNA (dsDNA) was studied. The results showed that well-organized dsDNA monolayers can distinguish the electron spin with high spin polarization at room temperature. Furthermore, the spin polarization increases with the dsDNA length and no spin polarization exists in single-stranded DNA (ssDNA). This finding is surprising since the DNA molecule is nonmagnetic and has small spin-orbit coupling that could not support such high spin selectivity.
Very recently, Dr. Ai-Min Guo and Prof. Qing-feng Sun, in Beijing National Laboratory for Condensed Matter Physics and Institute of Physics, proposed a model Hamiltonian to explain the above-mentioned experiments. This model Hamiltonian includes the environment-induced dephasing, the spin-orbit coupling, and the double-helix structure of the DNA molecule. The structure of the dsDNA is illustrated in Figure 1. Based on this Hamiltonian, they investigated the quantum spin transport through single dsDNA and ssDNA connected to nonmagnetic electrodes. Their results indicated that the electrons transmitted through the dsDNA are highly polarized and the spin polarization increases with the dsDNA length (see Figure 3). In addition, they found that no spin polarization exists for the ssDNA. These results are in good agreement with the experiments. The physical mechanism is attributed to the combination of the dephasing, the spin-orbit coupling, and the chiral symmetry. No spin polarization occurs if any aforementioned factor is absent (see Figure 2). Moreover, they found that the spin polarization could be improved by decreasing the helix angle. These results may be beneficial for designing DNA-based spintronic devices. This work was published in Physical Review Letters 108, 218102 (2012).
This work was supported by Chinese Academy of Sciences, the National Natural Science Foundation of China, and the ministry of science and technology of China.
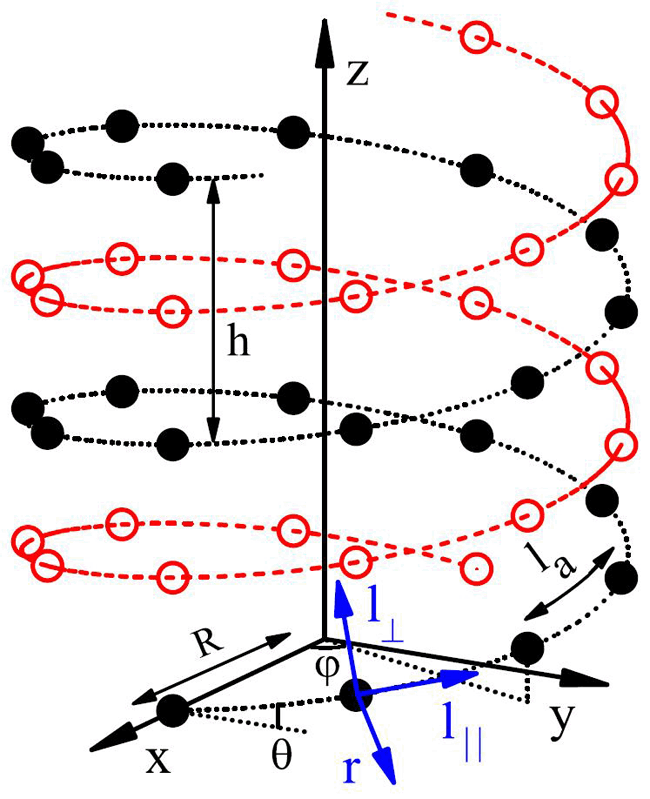 |
Figure 1. Schematic view of double-stranded DNA. (Image by Qing-feng Sun et al.) |
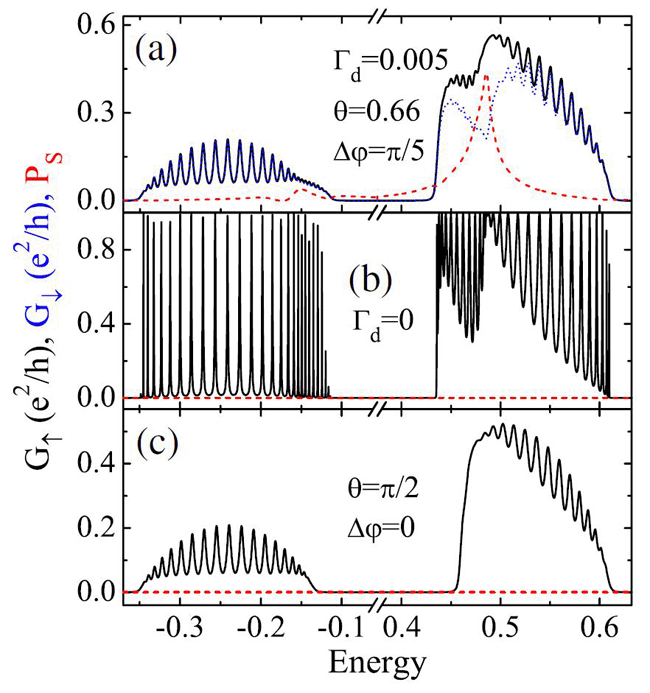 |
Figure 2. (a) Energy dependence of the conductances for spin-up and spin-down electrons and the spin polarization for realistic situation. (b) and (c) show the conductance for spin-up electron and the spin polarization in the absence of the dephasing and of the helical symmetry, respectively. (Image by Qing-feng Sun et al.) |
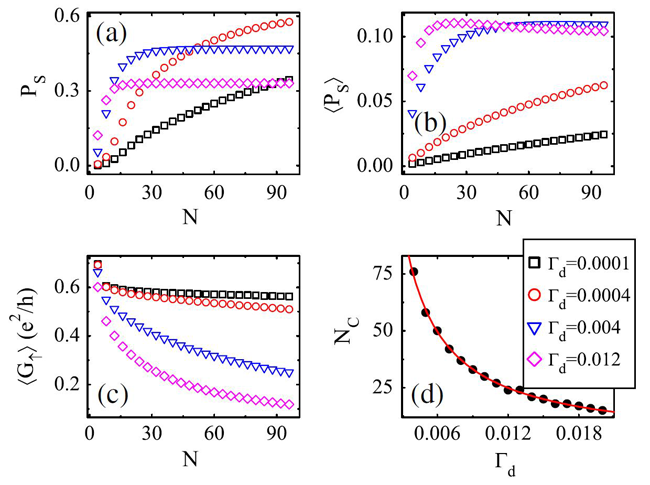 |
Figure 3. Length dependence of (a) the spin polarization, of (b) the averaged spin polarization, and of (c) the averaged conductance for spin-up electron. (d) The critical length vs the dephasing parameter. (Image by Qing-feng Sun et al.) |